The human central nervous system is extremely complex, consisting of about 86 billion neurons,1 and with each neuron capable of forming thousands of connections with other neurons. It is these connections that make the nervous system capable of processing information, but up until the mid-20th century some key aspects of their functions were unknown. NHMRC-funded researchers made critical breakthroughs in our understanding of how neurons communicate with each other, and in doing so provided a foundation for modern neuroscience and neurotransmitter-based medicine.
Origin
The idea that the brain is responsible for functions such as memory, cognition and willed action became part of medical knowledge at least as early as the second century CE.2 Over the course of history, too, it had become widely understood that nerves connected different body-parts to the brain. But it was not clear how the brain and nerves worked.
In 1873, individual nerve cells (neurons) were visualised under a microscope for the first time, revealing that they formed complex networks. Unlike the types of cells making up other body organs, neurons were found to possess long fibres (up to 1 metre), called axons, which they used to connect to, and send signals to, many other neurons.
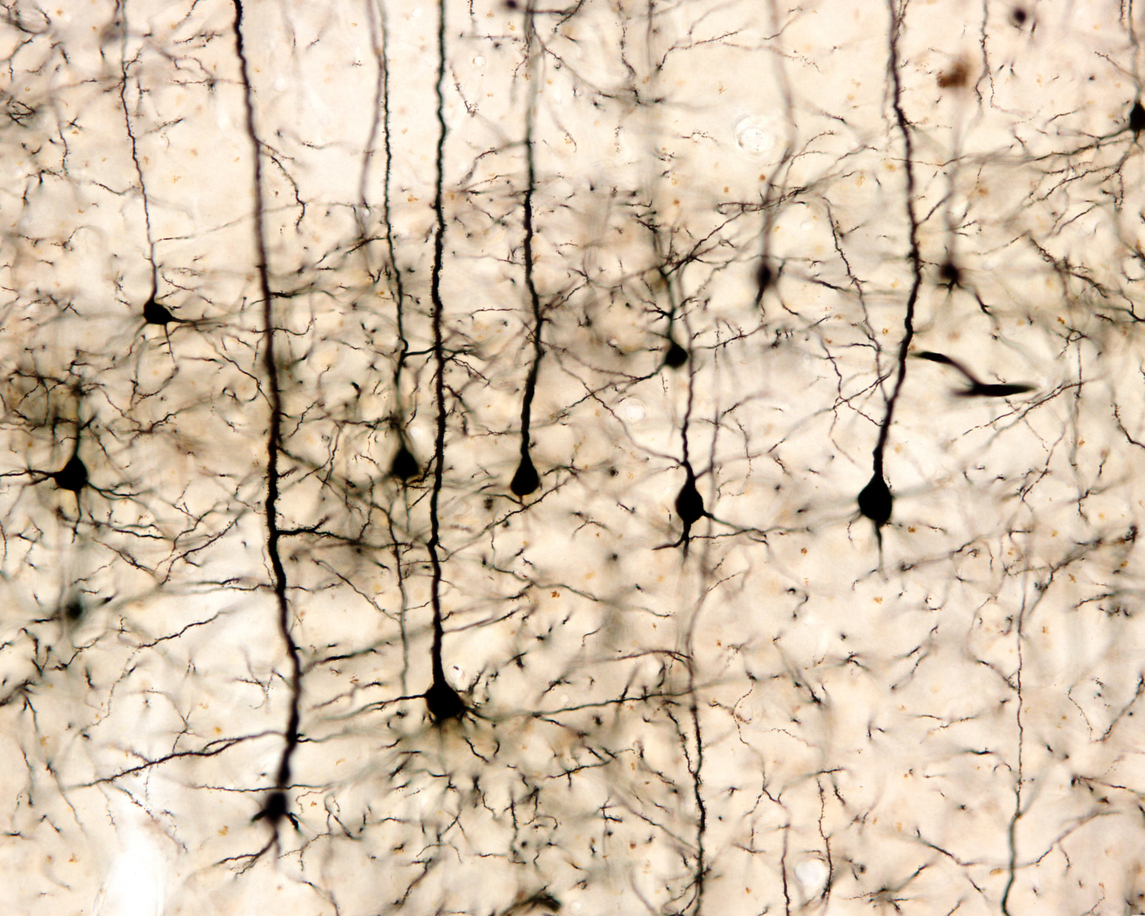
At the connection points between one neuron and another, or between neurons and muscle and glandular cells, it was inferred that there was a very small gap – so small, in fact, that it was not able to be visualised using a light microscope.
Oxford neuroscientist Charles Sherrington called these points of connection ‘synapses’ and in his 1906 book The Integrative Action of the Nervous System he hypothesized that the way neurons transmitted signals along their own axons, which was known to involve the flow of electrical currents, might not be the same as the way that signals were communicated across the synapse.
Sherrington also noted that the nervous system could only function because of a complex interaction of excitation (sending signals) and inhibition (preventing signals from being sent). If this were not true then, for example, two opposing muscles could each be trying to operate simultaneously against the actions of the other. Because inhibition could only be occurring at the synapse, understanding synaptic transmission was key to understanding nervous system functioning.
During the first half of the 20th century, the nature of synaptic transmission was an open question, and the source of considerable debate among neuroscientists. Some thought that the process was chemical, others thought it was electrical. Resolving this question was vital because very little substantive progress could be made in neuroscience until an answer was found.
After reading Sherrington’s book and realising that so little was known about the brain, University of Melbourne medical student John Eccles resolved to become a neuroscientist. He gained a scholarship to work with Sherrington and, after completing a residency at St Vincent’s Hospital, travelled to Oxford in 1925.
Investment
During the period 1925-1935, Eccles worked in Sherrington’s laboratory. He then accepted an appointment as Director of the Kanematsu Memorial Institute of Pathology at Sydney Hospital. During the years 1938 to 1944, the research efforts of Eccles and his colleagues Bernard Katz and Stephen Kuffler were supported by a succession of NHMRC grants.
Funding to support Eccles’ research was later provided by the University of Otago and the Medical Research Council of New Zealand. It was also provided by the Australian Government, through its support for the John Curtin School of Medical Research (JCSMR) at the Australian National University (ANU).
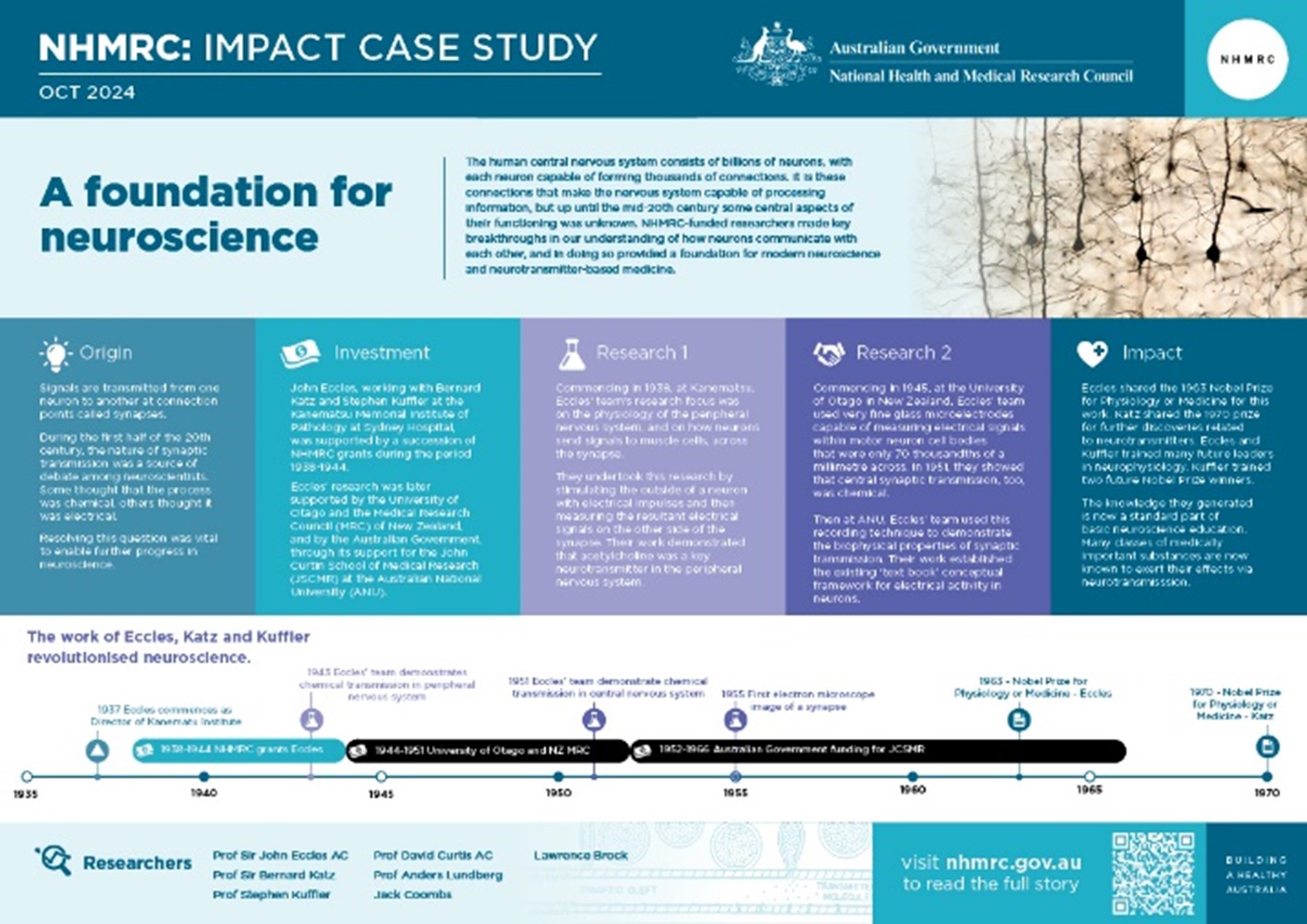
The PDF poster version of this case study includes a graphical time showing NHMRC grants provided and other events described in the case study.
Research
Early in the chemical/electrical debate, Eccles had come to the conclusion that synaptic transmission must be electrical because it seemed too rapid to be chemical.3, 4
The initial focus of the Kanematsu team’s research was on the physiology of the peripheral nervous system, and on how neurons send signals to muscle cells, across the synapse. They undertook this research by stimulating the outside of a neuron with electrical impulses and then measuring the resultant electrical signals on the other side of the synapse. This work demonstrated that a chemical (acetylcholine) was responsible for neurotransmission at the neuro-muscular synapse.4
However, Eccles and many other researchers still held the view that synapses in the central nervous system (such as, in the brain and spinal cord) were electrical rather than chemical. This was because the electrical signals being measured by researchers appeared similar regardless of whether measurement took place across the synapse or along an axon.4, 5
In 1945, having commenced work at the University of Otago in New Zealand, Eccles noted that ‘the electrical hypothesis has had the grave defect that . . . it has never been stated in such precise terms that it could be subjected to crucial tests’.6 Eccles resolved to create such a test. In 1947, he published an electrical theory of synaptic inhibitory action which appeared to conform with all of the available experimental evidence.7
Working with Otago University electronic engineer colleague Jack Coombs and medical graduate Lawrence Brock, Eccles began to test this theory using glass microelectrodes capable of recording voltage changes within (as opposed to outside) spinal cord motor neurons.
The world-leading technique that they developed involved inserting electrodes within motor neuron cell bodies.8 These were only 70 micrometres (thousandths of a millimetre) across so, to perform the experiment, very fine electrodes were necessary. These were based upon thin glass capillary tubes that tapered to a very small opening at one end, allowing them to enter the cell body without causing it much damage. Eccles, Coombs and Brock used this apparatus, plus a recording device (the Electronic Stimulating and Recording Unit or ESRU, developed by Coombs) which was ‘unmatched in neurophysiology laboratories elsewhere’.9
In mid-1951, the team performed an experiment which showed, contrary to Eccles’ theory, that central synaptic transmission must be chemical in nature. Consequently, he abandoned the electrical hypothesis. Because Eccles had been a leader of the ‘electrical’ camp of neuroscientists, once he changed his position, broader opposition to the concept of chemical transmission gradually waned during the 1950s.8
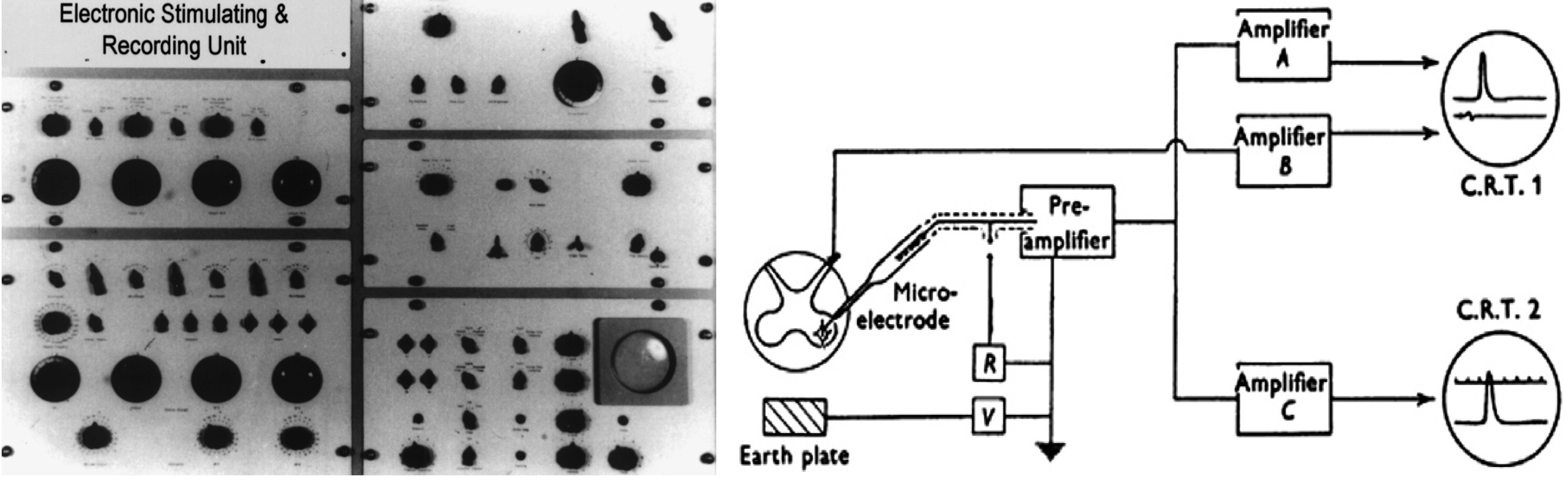
Later, at the JCSMR, Eccles and colleagues used the intracellular recording technique to demonstrate the ionic mechanisms underlying excitation and inhibition at spinal synapses. This work, which centred on the biophysical properties of synaptic transmission, established the current ‘textbook’ conceptual framework for the ionic mechanisms of membrane activity.4
Translation
Over time, some of the reasons why synaptic transmission is so complex started to become clear. In the first place, the space between the pre- and postsynaptic cells (called the synaptic cleft) was found to vary in width. At its narrowest the cleft might be only 20 nanometres (millionths of a millimetre) wide, although it could be twice this width, and its width influenced how quickly synaptic transmission could occur. Proof that a synaptic cleft existed was only available from 1955 when the first electron microscopy image of a vertebrate’s central nervous system synapse was published.10
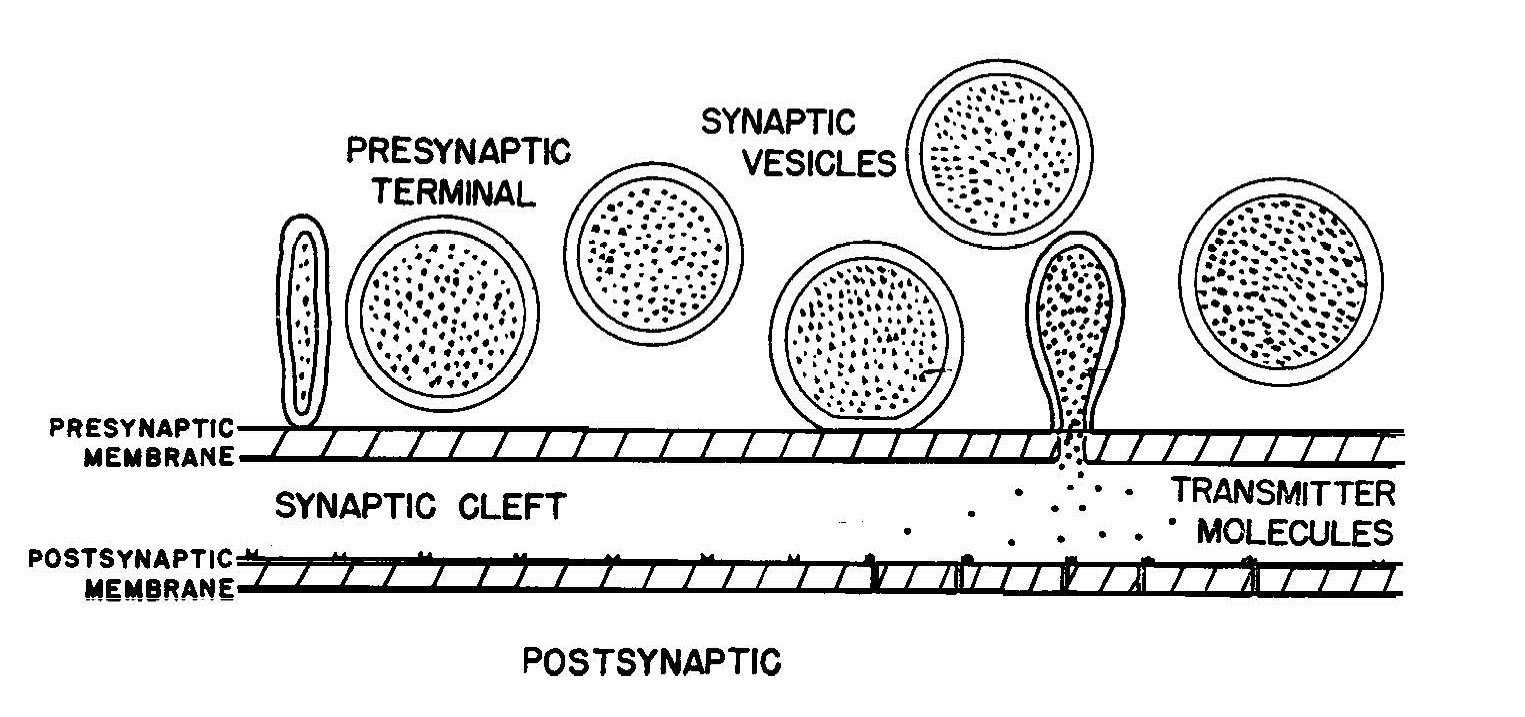
Credit: Nobel Prize Organisation
Neurons were found to be either exclusively excitatory or exclusively inhibitory, depending upon the particular type of neurotransmitter they released. However, some types of neurons could release several different types of neurotransmitters from a single synapse, and these might have slower- or faster-acting effects.
Moreover, later work found special types of synapses called ‘gap junctions’ that physically connect some types of neurons together, enabling electrical transmission between these cells. Thus, Eccles’ early idea favouring electrical synapses was correct in some regions of the nervous system.
Outcomes and impacts
Eccles, Katz, and Kuffler all had distinguished careers that literally changed the shape of neuroscience.
Eccles was awarded the 1963 Nobel Prize for Physiology or Medicine for his work. He shared the prize with Alan Hodgkin and Andrew Huxley, in Cambridge, who had shown how electrical signals pass down the axon to arrive at the synapse.
Katz shared the 1970 Nobel Prize for Physiology or Medicine for the discovery that neurotransmitter release at synapses is ‘quantal’, meaning that at any particular synapse, the amount of neurotransmitter released is never less than a certain amount and, if more, is always an integral number of times this amount. Scientists now understand that this circumstance arises because, prior to their release into the synaptic cleft, transmitter molecules reside in similar-sized subcellular packages known as synaptic vesicles, which can fuse with the membrane to release their contents.
Eccles and Kuffler trained many future leaders in neurophysiology. Kuffler trained two future Nobel Prize winners.11, 12 During Eccles’ 14 years at ANU there were, in the JCSMR Department of Physiology, 74 research workers from 20 different countries. Of these, 41 from 14 countries collaborated and published with Eccles.13
When Eccles began his research in 1925, the world’s production of neuroscience literature was ‘unbelievably small, so that one could easily survey the total publications’.14 Following the work of Eccles and his colleagues, publications reporting research related to neurotransmitters expanded from a handful each year to thousands.
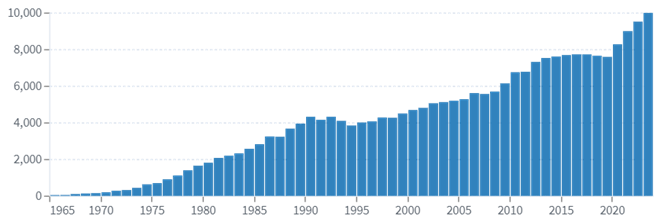
Source: lens.org
Many different classes of medically-important substances are now known to exert their effects via neurotransmission. These include:
- stimulants such as caffeine, nicotine, amphetamines and cocaine
- sedatives such as alcohol and cannabis and tranquilisers such as benzodiazepines
- anaesthetics, including opioids such as morphine and codeine
- antipsychotics such as chlorpromazine
- antidepressants
- psychedelic drugs such as LSD and ecstasy
- neurotoxins including botulism and tetanus toxins, and snake and spider venoms.
Although chlorpromazine and other drugs acting on the central nervous system were in use before the 1950s, it was only in the 1960s that the mechanism of these agents was understood in terms of their action on chemical neurotransmitters or their receptors. Neurotransmitters are now an integral part of brain science and clinical neurology.8
Improved understanding of neurotransmitters has allowed medicines to be used that target particular types of neurotransmitter systems within the brain. Neurotransmitter-based medicines have been approved for use for major depressive disorder, for postpartum depression, and for multiple sclerosis.15
An understanding of neurotransmitters has been vital for improving treatments for epilepsy, which is the most common chronic brain disorder, affecting 50 million people of all ages worldwide.16 Epileptic seizures occur when there is an imbalance of the excitatory and inhibitory circuits in the brain. Neurotransmitter-based medications, which decrease excitation or enhance inhibition, are particularly important for treating epilepsy.
Researchers
Professor Sir John Eccles AC
John Carew Eccles (1903-1997) graduated from the University of Melbourne in Medicine with first class honours in 1925, and as Victorian Rhodes Scholar for 1925 entered Magdalen College, Oxford, as an undergraduate. In 1927, he gained first class honours in Natural Sciences, the Christopher Welch Scholarship and a Junior Research Fellowship at Exeter College, Oxford. From 1928 to 1931 he was research assistant to Sherrington. He was awarded an Oxford DPhil degree in 1929 for a thesis on excitation and inhibition. Later Oxford appointments were to a Staines Medical Fellowship at Exeter College in 1932, a tutorial fellowship of Magdalen College, and a University Demonstratorship in 1934. Eccles was Director of the Kanematsu Institute (1937-1943), Professor of Physiology at the University of Otago, New Zealand (1944-1951) and Professor of Physiology at the ANU (1952-1966), and also worked at the Feinberg School of Medicine at Northwestern University in Chicago (1966-1968). He was professor at The State University of New York at Buffalo from 1968 until he retired in 1975.
Eccles was appointed a Knight Bachelor in 1958 in recognition of services to physiological research. He won the Australian of the Year Award in 1963 and in that same year shared the Nobel Prize for Physiology or Medicine. In 1990 he was appointed a Companion of the Order of Australia (AC).
Professor Sir Bernard Katz
Bernard Katz (1911-2003) studied medicine at the University of Leipzig, graduating in 1934. He completed a PhD at University College London (UCL) in 1938 then won a fellowship to work with Eccles at the Kanematsu Institute. During the Second World War he worked as a radar officer in the Royal Australian Air Force then moved back to UCL, where he was made professor in 1952 and head of the Biophysics Department, where he remained until 1978.
Katz was awarded the Royal Society Copley Medal (1967), shared the Nobel Prize in Physiology or Medicine (1970), and was awarded the Ralph W. Gerard Prize in Neuroscience (1990).
Professor Stephen Kuffler
Stephen William Kuffler (1913-1980) completed medical studies in Vienna in 1937 and was then invited by Eccles to move to the Kanematsu Institute. After the war he moved to the University of Chicago and then to Johns Hopkins University Medical School. He also contributed to research at the Woods Hole Marine Biological Laboratory. In 1959, Kuffler moved to the Department of Pharmacology at Harvard Medical School. From 1966 to 1974, Kuffler was the Robert Winthrop Professor of Neurobiology at Harvard and in 1974 he became the John Franklin Enders University Professor. In 1971, he was made a member of the Royal Society and in 1972 he was awarded the Louisa Gross Horwitz Prize from Columbia University.
Professor David Curtis AC
David Roderick Curtis (1927-2017) completed a medical degree at the University of Melbourne in 1950, was a resident medical officer at the Royal Melbourne Hospital (1951-1953) and registrar at the Department of Neurology, The Alfred Hospital, Melbourne (1953-1954) before moving to the ANU. He completed a PhD in 1957, was a research fellow within the Department of Physiology (1954-1966) and was founding Professor of Pharmacology/ Neuropharmacology at JCSMR from 1958. He was Director of JCSMR (1989-1992).
Curtis was a Fellow of The Royal Society, London (1974), Vice-President and then President of the Australian Academy of Science (1974-1977, 1986-1990) and received the Academy’s Macfarlane Burnet Medal and Lecture (1983). He was President, Australian Physiological and Pharmacological Society (1991-1995), was appointed a Companion of the Order of Australia (1992) and was awarded the Centenary Medal (2001).
Professor Anders Lundberg
Anders Lundberg (1920-2009) studied medicine at Lund University, Sweden, graduating in 1947. He then undertook neuroscience at the Karolinska Institute. After completing a PhD in 1948, he continued research at the Rockefeller Institute in New York, then returned to Lund as an Associate Professor. In 1957, he was invited by Eccles to join the JCSMR team where he worked until 1961, when he became Professor of Physiology at the University of Gothenburg where he worked until retirement in 1986. However, he continued contributing to research publications until 2000.
Lundberg was awarded several prizes, including the Alvarenga Prize (1963), the Eric K. Fernström Nordic Prize in Medicine (1978) and the Wakeman Award for research of relevance for spinal cord injury from Duke University (1986). He was elected foreign member of the Royal Danish and Norwegian Academies of Sciences (1976 and 1981) and the French Academy of Sciences (1989).
Jack Coombs
John (Jack) S Coombs (1917-1993) completed a Bachelor of Science (1938) and Master of Science (1940) in physics at the University of Otago where he then held faculty appointments as an Assistant Lecturer (1940–1946), Lecturer (1947–1950), and part-time lecturer (1951) in Physics. He was appointed a Fellow at JCSMR in 1952, and Electronics Engineer in 1961. At JCSMR, from 1952 until his retirement in 1977, he continued to design and make innovative electronic equipment for the Departments of Physiology and Pharmacology.
Lawrence Brock
Lawrence G Brock (1923-1996) completed a Bachelor of Science in mathematics, chemistry and physics (1944) at the Auckland University College and an undergraduate degree in medicine and surgery (1949) at the University of Otago in Dunedin. He was appointed as an Assistant Lecturer (1950–1952) to the Otago Department of Physiology. In 1953–1954, he was awarded a Nuffield Commonwealth Travelling Fellowship to undertake further neuroscience training at the University of Cambridge Returning to Dunedin, he was appointed Senior Lecturer in the Department of Physiology (1955–57). He then changed his career focus to surgery and in 1961 he became a Fellow of the Royal College of Surgeons in the UK. He became a lecturer in the Nuffield Department of Surgery, University of Oxford (1962–68) then switched to diagnostic radiology at Oxford (1967–72) and neuroradiology (Walton Hospital, Liverpool, 1972– 1989).
Partners
This case study was developed with input from Professor John Bekkers, Head of the Eccles Institute of Neuroscience in the John Curtin School of Medical Research at ANU.
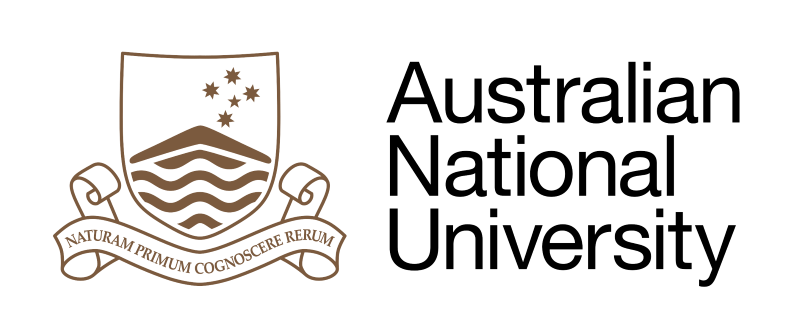
References
The information and images from which NHMRC Impact Case Studies are produced may be obtained from a number of sources including our case study partner, NHMRC’s internal records and publicly available materials. Key sources of information consulted for this case study include:
1 Azevedo FA, Carvalho LR, Grinberg LT, Farfel JM, Ferretti RE, Leite RE, Jacob Filho W, Lent R, Herculano-Houzel S. Equal numbers of neuronal and nonneuronal cells make the human brain an isometrically scaled-up primate brain. J Comp Neurol. 2009 Apr 10;513(5):532-41
2 Freemon FR. Galen's ideas on neurological function. J Hist Neurosci. 1994 Oct;3(4):263-71
3 Eccles JC. The spinal cord and reflex action. Annual Review of Physiology. 1939 Mar;1(1):363-84
4 Todman D. John Carew Eccles (1903-1997). J Neurol. 2008 Jun;255(6):946-7
5 Fulton J (1949): Physiology of the Nervous System. New York, Oxford University Press, 3rd edition
6 Eccles JC. An electrical hypothesis of synaptic and neuromuscular transmission. Nature. 1945 Dec 8;156(3971):680-3
7 Brooks CM, Eccles JC. An electrical hypothesis of central inhibition. Nature. 1947 Jun 7;159(4049):760-4
8 Kuffler SW. Reviews. Journal of Neurophysiology. 1957 Sep;20(5):549-551
9 Todman D. John Eccles (1903-97) and the experiment that proved chemical synaptic transmission in the central nervous system. J Clin Neurosci. 2008 Sep;15(9):972-7
10 Sanchez Avila A, Henstridge CM. Array tomography: 15 years of synaptic analysis. Neuronal Signal. 2022 Sep 23;6(3)
11 Horn JP. The heroic age of neurophysiology. Hospital Practice. 1992 Jul 15;27(7):65-74
12 Akkermans R. Stephen Kuffler. The Lancet Neurology. 2017 Jan 1;16(1):30
13 Curtis DR, Andersen P. John Carew Eccles 1903–1997. Hist Rec Aust Sci. 2001a; 13:439–473
14 Eccles JC. My scientific odyssey. Annu Rev Physiol. 1977;39:1-18
15 Urquhart L. FDA new drug approvals in Q1 2019. Nature Reviews Drug Discovery. 2019 Apr 10:NA-
16 World Health Organisation. Epilepsy. Source: https://www.who.int/health-topics/epilepsy. Accessed 19 September 2024